The computing world has been chasing the elusive “quantum supremacy” title for quite some time, but it looks as though new developments have pushed us even closer to achieving that goal today.
In October 2019, Google announced that Sycamore, its quantum computer, had solved an extremely complex equation that would be virtually impossible to solve by a traditional computer. The equation, which experts estimate would take the world’s most powerful supercomputers 10,000 years to solve, was solved by Sycamore in 200 seconds.
Though the solution that Sycamore arrived at has little practical application outside of the quantum mechanics world (it’s the equivalent of generating a random list of numbers and checking them 1 million times), it does, however, offer some big implications for improvements in processing power.
So then, what exactly is a quantum computer, how does it work, and what makes it superior to other computers? Can quantum computers beat supercomputers? We’ll look at some of the recent developments and explore how quantum computing might change the way computers work.
Quantum Computers: How They Work
Regular computers use bits of information to perform various calculations; like a simple on and off switch, they only exist as 0 or 1 at any particular moment. Because of the nature of quantum mechanics, a qubit can be both 0 and 1 at the same time, allowing an exponential increase in processing power. If you have two bits, then those bits can only store one each of four possible combinations of 0 and 1 at once (which would be either 00, 01, 10, or 11). However, two qubits could store all four of these options at once.
With more qubits added, the processing power of the computer will increase exponentially. Google’s quantum computer, Sycamore, has 53 qubits at its disposal, which translates to around 10,000,000,000,000,000, or 10 quadrillion, different combinations.
In addition to the use of qubits, quantum computers also benefit from the quantum properties known as “quantum entanglement” and “superpositioning.” Quantum entanglement is a phenomenon in which particles that have interacted at one point can become entangled. When this happens, it is possible to know the state of another particle by measuring the state of one it is entangled with, no matter how much distance is between them. For quantum computing, this means you can know the state of all a quantum computer’s qubits simultaneously if they are entangled.
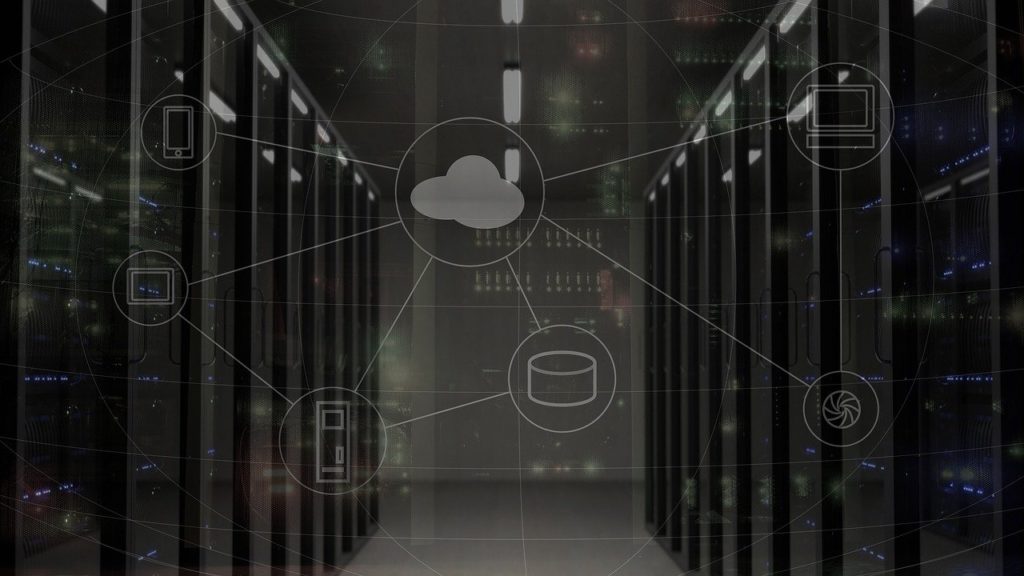
Problems with Quantum Computing
The superiority of quantum computers to regular computers seems obvious at first glance, but quantum computers have their share of problems. Those qubits that become entangled can also become unentangled relatively easily and quickly, making them susceptible to errors or noise as a result. Traditional computers also have the ability to store millions upon millions of bits inside of their processors, but quantum computers have trouble scaling the number of qubits that can be used. Although there has been significant progress in the world of quantum computing, we are still not quite there in terms of consistent results and practical applications.
Latest Advancements
Recent advancements in quantum computing are helping drive us closer toward practical applications for quantum computers. Some of that advancement has recently come via researchers at Princeton University, with results published on December 25, 2019. Most current quantum computers use superconducting circuits, but these elements can be quite expensive.
The Princeton team looked at silicon spin qubits for quantum computers, which have the potential to be much cheaper than superconducting elements since traditional computers already use silicon. One of the problems with silicon spin qubits has been their inability to communicate with each other unless they are directly next door, which results in information having to be passed from qubit to qubit over great distances.
The Princeton University researchers recently beat this limitation, demonstrating that silicon spin qubits can now communicate across greater distances when placed far apart (relatively speaking) on computer chips.
Silicon spin qubits have several important advantages over other types, including their relatively low cost of manufacturing and their ability to retain a quantum state for longer than their competitors. The biggest issue with these has been the connection between different silicon spin qubits. The team of Princeton researchers has connected the qubits using a “wire,” which is essentially a very small cavity containing just a single particle of light. This particle, called a photon, carries information from one qubit to another. In order to make this information exchange work, both qubits and the photon must all be vibrating at the same frequency.
By achieving this step, researchers have provided an important proof-of-possibility that will help to facilitate an important level of flexibility for silicon spin qubits in terms of how to lay them out and wire them in the quantum microchips of the future.